Classification and Types of Energy Recovery Systems
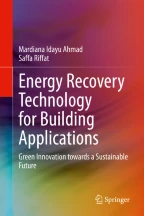
In general, energy recovery systems can be commonly identified based on their classification and types. They are classified into: (1) application, in terms of process-to-process system, process-to-comfort system and comfort-to-comfort system and (2) working mechanism in terms of air-to-air energy recovery, earth-to-air energy recovery, earth-to-water energy recovery. In the context of types, there are five common energy recovery systems, which can be found in the global market and have been extensively studied which are based on the construction of their heat exchangers. These include fixed-plate, rotary enthalpy wheel, heat pipe, run-around and thermosiphon. This chapter presents classifications and types of energy recovery systems as well as an overview of existing research in these domains.
This is a preview of subscription content, log in via an institution to check access.
Access this chapter
Subscribe and save
Springer+ Basic
€32.70 /Month
- Get 10 units per month
- Download Article/Chapter or eBook
- 1 Unit = 1 Article or 1 Chapter
- Cancel anytime
Buy Now
Price includes VAT (France)
eBook EUR 85.59 Price includes VAT (France)
Softcover Book EUR 105.49 Price includes VAT (France)
Hardcover Book EUR 105.49 Price includes VAT (France)
Tax calculation will be finalised at checkout
Purchases are for personal use only
References
- Abe, O. O., Simonson, C. J., Besant, R. W., & Shang, W. (2006). Effectiveness of energy wheels from transient measurements: Part II—Results and verification. International Journal of Heat and Mass Transfer, 49(1), 63–77. https://doi.org/10.1016/j.ijheatmasstransfer.2005.08.009ArticleGoogle Scholar
- Abu-Khader, M. (2012). Plate heat exchangers: Recent advances. Renewable and Sustainable Energy Reviews, 16, 1883. https://doi.org/10.1016/j.rser.2012.01.009ArticleGoogle Scholar
- Abu-Mulaweh, H. I. (2006). Design and performance of a thermosiphon heat recovery system. Applied Thermal Engineering, 26(5), 471–477. https://doi.org/10.1016/j.applthermaleng.2005.08.003ArticleGoogle Scholar
- Ahmadzadehtalatapeh, M., & Yau, Y. H. (2011). The application of heat pipe heat exchangers to improve the air quality and reduce the energy consumption of the air conditioning system in a hospital ward—A full year model simulation. Energy and Buildings, 43(9), 2344–2355. https://doi.org/10.1016/j.enbuild.2011.05.021ArticleGoogle Scholar
- Akbari, S., Hemingson, H. B., Beriault, D., Simonson, C. J., & Besant, R. W. (2012a). Application of neural networks to predict the steady state performance of a run-around membrane energy exchanger. International Journal of Heat and Mass Transfer, 55(5), 1628–1641. https://doi.org/10.1016/j.ijheatmasstransfer.2011.11.019ArticleMATHGoogle Scholar
- Akbari, S., Simonson, C. J., & Besant, R. W. (2012b). Application of neural networks to predict the transient performance of a run-around membrane energy exchanger for yearly non-stop operation. International Journal of Heat and Mass Transfer, 55(21), 5403–5416. https://doi.org/10.1016/j.ijheatmasstransfer.2012.04.033ArticleGoogle Scholar
- Al-Ajmi, F., Loveday, D. L., & Hanby, V. I. (2006). The cooling potential of earth–air heat exchangers for domestic buildings in a desert climate. Building and Environment, 41(3), 235–244. https://doi.org/10.1016/j.buildenv.2005.01.027ArticleGoogle Scholar
- Al-Zubaydi, A., & Hong, G. (2018). Experimental investigation of counter flow heat exchangers for energy recovery ventilation in cooling mode. International Journal of Refrigeration, 93, 132–143. https://doi.org/10.1016/j.ijrefrig.2018.07.008ArticleGoogle Scholar
- Anisimov, S., Jedlikowski, A., & Pandelidis, D. (2015). Frost formation in the cross-flow plate heat exchanger for energy recovery. International Journal of Heat and Mass Transfer, 90, 201–217. https://doi.org/10.1016/j.ijheatmasstransfer.2015.06.056ArticleGoogle Scholar
- ASHRAE (Ed.). (2005). ASHRAE handbook of fundamentals. Atlanta, GA: American Society of Heating, Refrigerating and Air-Conditioning Engineers, Inc.. Google Scholar
- Bordoloi, N., Sharma, A., Nautiyal, H., & Goel, V. (2018). An intense review on the latest advancements of earth air heat exchangers. Renewable and Sustainable Energy Reviews, 89, 261–280. https://doi.org/10.1016/j.rser.2018.03.056ArticleGoogle Scholar
- Burlacu, A., Sosoi, G., Vizitiu, R. Ș., Bărbuță, M., Lăzărescu, C. D., Ciocan, V., & Șerbănoiu, A. A. (2018). Energy efficient heat pipe heat exchanger for waste heat recovery in buildings. Procedia Manufacturing, 22, 714–721. https://doi.org/10.1016/j.promfg.2018.03.103
- Calautit, J. K., & Hughes, B. R. (2016). A passive cooling wind catcher with heat pipe technology: CFD, wind tunnel and field-test analysis. Applied Energy, 162, 460–471. https://doi.org/10.1016/j.apenergy.2015.10.045ArticleGoogle Scholar
- Chaudhry, H. N., Calautit, J. K., & Hughes, B. R. (2017). Optimisation and analysis of a heat pipe assisted low-energy passive cooling system. Energy and Buildings, 143, 220–233. https://doi.org/10.1016/j.enbuild.2017.02.002ArticleGoogle Scholar
- Chel, A., & Tiwari, G. N. (2010). Stand-alone photovoltaic (PV) integrated with earth to air heat exchanger (EAHE) for space heating/cooling of adobe house in New Delhi (India). Energy Conversion and Management, 51(3), 393–409. https://doi.org/10.1016/j.enconman.2009.10.001ArticleGoogle Scholar
- Chen, L., & Yu, Z. (2017). Research of a rotary desiccant wheel based hybrid air conditioning system with natural cold source. Procedia Engineering, 205, 492–496. https://doi.org/10.1016/j.proeng.2017.10.400ArticleGoogle Scholar
- Dallaire, J., Gosselin, L., & da Silva, A. K. (2010). Conceptual optimization of a rotary heat exchanger with a porous core. International Journal of Thermal Sciences, 49(2), 454–462. https://doi.org/10.1016/j.ijthermalsci.2009.07.027ArticleGoogle Scholar
- Dhital, P., Besant, R. W., & Schoenau, G. J. (1995). Integrating run-around heat exchanger systems into the design of large office buildings. ASHRAE Transactions, 101, 979–991. Google Scholar
- Diao, Y. H., Liang, L., Kang, Y. M., Zhao, Y. H., Wang, Z. Y., & Zhu, T. T. (2017). Experimental study on the heat recovery characteristic of a heat exchanger based on a flat micro-heat pipe array for the ventilation of residential buildings. Energy and Buildings, 152, 448–457. https://doi.org/10.1016/j.enbuild.2017.07.045ArticleGoogle Scholar
- Emerson, W. H. (1983). Designing run-around coil systems. Journal of Heat Recovery Systems, 3(4), 305–309. https://doi.org/10.1016/0198-7593(83)90011-5ArticleGoogle Scholar
- Ersöz, M. A., & Yıldız, A. (2016). Thermoeconomic analysis of thermosyphon heat pipes. Renewable and Sustainable Energy Reviews, 58, 666–673. https://doi.org/10.1016/j.rser.2015.12.250ArticleGoogle Scholar
- Fan, H., Simonson, C., Besant, R. W., & Shang, W. (2005). Run-around heat recovery system using cross-flow flat-plate heat exchangers with aqueous ethylene glycol as the coupling fluid. ASHRAE Transactions, 111, 901–910. Google Scholar
- Fan, H., Simonson, C., Besant, R., & Shang, W. (2006). Performance of a run-around system for HVAC heat and moisture transfer applications using cross-flow plate exchangers coupled with aqueous lithium bromide. HVAC&R Research, 12, 313–336. https://doi.org/10.1080/10789669.2006.10391181ArticleGoogle Scholar
- Fernandez-Seara, J., Diz, R., Uhia, F. J., Dopazo, A., & Ferro, J. M. (2011). Experimental analysis of an air-to-air heat recovery unit for balanced ventilation systems in residential buildings. Energy Conversion and Management, 52(1), 635–640. https://doi.org/10.1016/j.enconman.2010.07.040ArticleGoogle Scholar
- Forsyth, B. I., & Besant, R. W. (1988). The performance of a run-around heat recovery system using aqueous glycol as a coupling liquid. ASHRAE Transactions, 94, 532–545. Google Scholar
- Ge, G., Moghaddam, DG., Namvar, R., Simonson, CJ., Besant, RW. (2013). Analytical model based performance evaluation, sizing and coupling flow optimization of liquid desiccant run-around membrane energy exchanger systems. Energy and Buildings, 62, 248–257. Google Scholar
- Gherasim, I., Taws, M., Galanis, N., & Nguyen, C. (2011). Heat transfer and fluid flow in a plate heat exchanger part I. Experimental investigation. International Journal of Thermal Sciences, 50, 1492–1498. https://doi.org/10.1016/j.ijthermalsci.2011.03.018ArticleGoogle Scholar
- Ghodsipour, N., & Sadrameli, M. (2003). Experimental and sensitivity analysis of a rotary air preheater for the flue gas heat recovery. Applied Thermal Engineering, 23(5), 571–580. https://doi.org/10.1016/S1359-4311(02)00226-0ArticleGoogle Scholar
- Han, H., Choo, Y.-B., & Kwon, Y.-I. (2007). An experimental study on the effect of outdoor temperature and humidity conditions on the performance of a heat recovery ventilator. Proceedings of Clima 2007 WellBeing Indoors. http://www.inive.org/members_area/medias/pdf/Inive%5Cclima2007%5CA07%5CA07Q1533.pdf
- Hemingson, H. B., Simonson, C. J., & Besant, R. W. (2011). Steady-state performance of a run-around membrane energy exchanger (RAMEE) for a range of outdoor air conditions. International Journal of Heat and Mass Transfer, 54(9), 1814–1824. https://doi.org/10.1016/j.ijheatmasstransfer.2010.12.036ArticleMATHGoogle Scholar
- Hemzal, K. (2006). Rotary heat exchanger efficiency influenced by air tightness. In: 17th air-conditioning and ventilation conference, Praha. Google Scholar
- Jafarinejad, T., Shafii, M. B., & Roshandel, R. (2019). Multistage recovering ventilated air heat through a heat recovery ventilator integrated with a condenser-side mixing box heat recovery system. Journal of Building Engineering, 24, 100744. https://doi.org/10.1016/j.jobe.2019.100744ArticleGoogle Scholar
- Jani, D. B., Mishra, M., & Sahoo, P. K. (2016). Experimental investigation on solid desiccant–vapor compression hybrid air-conditioning system in hot and humid weather. Applied Thermal Engineering, 104, 556–564. https://doi.org/10.1016/j.applthermaleng.2016.05.104ArticleGoogle Scholar
- Kappler, G., Dias, J. B., Haeberle, F., Wander, P. R., Moraes, C. A. M., & Modolo, R. C. E. (2019). Study of an earth-to-water heat exchange system which relies on underground water tanks. Renewable Energy, 133, 1236–1246. https://doi.org/10.1016/j.renene.2018.09.004ArticleGoogle Scholar
- Khan, T., Khan, M., & Ayub, Z. (2016). Single-phase flow pressure drop analysis in a plate heat exchanger. Heat Transfer Engineering, 38, 00. https://doi.org/10.1080/01457632.2016.1177430ArticleGoogle Scholar
- Kreith, F., & Bohn, M. S. (Eds.). (1997). Principles of heat transfer (Vol. 119, 7th ed.p. 187). Boston, MA: PWS Publishing Company. Google Scholar
- Lamb, B. R. (1982). Plate heat exchangers—A low-cost route to heat recovery. Journal of Heat Recovery Systems, 2(3), 247–255. https://doi.org/10.1016/0198-7593(82)90018-2ArticleGoogle Scholar
- Lin, S., Broadbent, J., & McGlen, R. (2005). Numerical study of heat pipe application in heat recovery systems. Applied Thermal Engineering, 25(1), 127–133. https://doi.org/10.1016/j.applthermaleng.2004.02.012ArticleGoogle Scholar
- Liu, X., & Tu, R. (2013). Performance comparison between enthalpy recovery wheels and dehumidification wheels. International Journal of Refrigeration, 36(8), 2308–2322. ArticleGoogle Scholar
- London, A. L., & Kays, W. M. (1951). The liquid-coupled indirect transfer regenerator for gas- turbine plants. ASME Transactions, 73, 529–542. Google Scholar
- Lu, Y., Wang, Y., Zhu, L., & Wang, Q. (2010). Enhanced performance of heat recovery ventilator by airflow-induced film vibration (HRV performance enhanced by FIV). International Journal of Thermal Sciences, 49(10), 2037–2041. https://doi.org/10.1016/j.ijthermalsci.2010.06.001ArticleGoogle Scholar
- Lu, T., Lü, X., Kibert, C., & Puttonen, J. (2016). The application of linear regression and the power law relationship of air-side heat transfer with field measurements to model the performance of run-around heat recovery systems. Energy and Buildings, 110, 453–467. https://doi.org/10.1016/j.enbuild.2015.10.028ArticleGoogle Scholar
- Mahmud, K., Mahmood, G. I., Simonson, C. J., & Besant, R. W. (2010). Performance testing of a counter-cross-flow run-around membrane energy exchanger (RAMEE) system for HVAC applications. Energy and Buildings, 42(7), 1139–1147. https://doi.org/10.1016/j.enbuild.2010.02.005ArticleGoogle Scholar
- Mardiana-Idayu, A., & Riffat, S. B. (2011). An experimental study on the performance of enthalpy recovery system for building applications. Energy and Buildings, 43(9), 2533–2538. https://doi.org/10.1016/j.enbuild.2011.06.009ArticleGoogle Scholar
- Mardiana-Idayu, A., & Riffat, S. B. (2012). Review on heat recovery technologies for building applications. Renewable and Sustainable Energy Reviews, 16(2), 1241–1255. https://doi.org/10.1016/j.rser.2011.09.026ArticleGoogle Scholar
- Min, J., & Su, M. (2010). Performance analysis of a membrane-based energy recovery ventilator: Effects of membrane spacing and thickness on the ventilator performance. Applied Thermal Engineering, 30(8), 991–997. https://doi.org/10.1016/j.applthermaleng.2010.01.010ArticleGoogle Scholar
- Nasif, M., Al-Waked, R., Morrison, G., & Behnia, M. (2010). Membrane heat exchanger in HVAC energy recovery systems, systems energy analysis. Energy and Buildings, 42(10), 1833–1840. https://doi.org/10.1016/j.enbuild.2010.05.020ArticleGoogle Scholar
- Nóbrega, C. E. L., & Brum, N. (2009). Modeling and simulation of heat and enthalpy recovery wheels. Energy, 34, 2063–2068. https://doi.org/10.1016/j.energy.2008.08.016ArticleGoogle Scholar
- O’Connor, D., Calautit, J., & Hughes, B. R. (2015). Effect of rotation speed of a rotary thermal wheel on ventilation supply rates of wind tower system. Energy Procedia, 75, 1705–1710. https://doi.org/10.1016/j.egypro.2015.07.432ArticleGoogle Scholar
- O’Connor, D., Calautit, J. K., & Hughes, B. R. (2016). A novel design of a desiccant rotary wheel for passive ventilation applications. Applied Energy, 179, 99–109. https://doi.org/10.1016/j.apenergy.2016.06.029ArticleGoogle Scholar
- O’Connor, D., Calautit, J. K., & Hughes, B. R. (2017). A novel design of a rotary desiccant system for reduced dehumidification regeneration air temperature. Energy Procedia, 142, 253–258. https://doi.org/10.1016/j.egypro.2017.12.040ArticleGoogle Scholar
- Patel, H., Ge, G., Abdel-Salam, M. R. H., Abdel-Salam, A. H., Besant, R. W., & Simonson, C. J. (2014). Contaminant transfer in run-around membrane energy exchangers. Energy and Buildings, 70, 94–105. https://doi.org/10.1016/j.enbuild.2013.11.013ArticleGoogle Scholar
- Persily, A. (1982). Evaluation of an air-to-air heat exchanger. Environment International, 8(1), 453–459. https://doi.org/10.1016/0160-4120(82)90063-0ArticleGoogle Scholar
- Rasouli, M., Akbari, S., Simonson, C. J., & Besant, R. W. (2014). Energetic, economic and environmental analysis of a health-care facility HVAC system equipped with a run-around membrane energy exchanger. Energy and Buildings, 69, 112–121. https://doi.org/10.1016/j.enbuild.2013.06.036ArticleGoogle Scholar
- Ruan, W., Qu, M., & Horton, W. T. (2012). Modeling analysis of an enthalpy recovery wheel with purge air. International Journal of Heat and Mass Transfer, 55(17), 4665–4672. https://doi.org/10.1016/j.ijheatmasstransfer.2012.04.025ArticleGoogle Scholar
- Ruivo, C. R., Angrisani, G., & Minichiello, F. (2015). Influence of the rotation speed on the effectiveness parameters of a desiccant wheel: An assessment using experimental data and manufacturer software. Renewable Energy, 76, 484–493. https://doi.org/10.1016/j.renene.2014.11.068ArticleGoogle Scholar
- San, J. Y., & Hsiau, S. C. (1993). Effect of axial solid heat conduction and mass diffusion in a rotary heat and mass regenerator. International Journal of Heat and Mass Transfer, 36(8), 2051–2059. https://doi.org/10.1016/S0017-9310(05)80136-XArticleGoogle Scholar
- Sauciuc, I., Akbarzadeh, A., & Johnson, P. (1995). Characteristics of two-phase closed thermosiphons for medium temperature heat recovery applications. Heat Recovery Systems and CHP, 15(7), 631–640. https://doi.org/10.1016/0890-4332(95)90043-8ArticleGoogle Scholar
- Sauer, H. J., & Howell, R. H. (1981). Promise and potential of air-to-air energy recovery systems. International Journal of Refrigeration, 4(4), 182–194. https://doi.org/10.1016/0140-7007(81)90049-9ArticleGoogle Scholar
- Seyed-Ahmadi, M., Erb, B., Simonson, C. J., & Besant, R. W. (2009a). Transient behavior of run-around heat and moisture exchanger system. Part IІ: Sensitivity studies for a range of initial conditions. International Journal of Heat and Mass Transfer, 52(25), 6012–6020. https://doi.org/10.1016/j.ijheatmasstransfer.2009.06.037ArticleMATHGoogle Scholar
- Seyed-Ahmadi, M., Erb, B., Simonson, C. J., & Besant, R. W. (2009b). Transient behavior of run-around heat and moisture exchanger system. Part І: Model formulation and verification. International Journal of Heat and Mass Transfer, 52(25), 6000–6011. https://doi.org/10.1016/j.ijheatmasstransfer.2009.07.012ArticleMATHGoogle Scholar
- Shabgard, H., Allen, M. J., Sharifi, N., Benn, S. P., Faghri, A., & Bergman, T. L. (2015). Heat pipe heat exchangers and heat sinks: Opportunities, challenges, applications, analysis, and state of the art. International Journal of Heat and Mass Transfer, 89, 138–158. https://doi.org/10.1016/j.ijheatmasstransfer.2015.05.020ArticleGoogle Scholar
- Shahsavar, A., & Khanmohammadi, S. (2019). Feasibility of a hybrid BIPV/T and thermal wheel system for exhaust air heat recovery: Energy and exergy assessment and multi-objective optimization. Applied Thermal Engineering, 146, 104–122. https://doi.org/10.1016/j.applthermaleng.2018.09.101ArticleGoogle Scholar
- Shao, L., & Riffat, S. B. (1997). Flow loss caused by heat pipes in natural ventilation stacks. Applied Thermal Engineering, 17(4), 393–399. https://doi.org/10.1016/S1359-4311(96)00029-4ArticleGoogle Scholar
- Shao, L., Riffat, S. B., & Gan, G. (1998). Heat recovery with low pressure loss for natural ventilation. Energy and Buildings, 28(2), 179–184. https://doi.org/10.1016/S0378-7788(98)00016-4ArticleGoogle Scholar
- Shen, S., Cai, W., Wang, X., Wu, Q., & Yon, H. (2016). Hybrid model for heat recovery heat pipe system in liquid desiccant dehumidification system. Applied Energy, 182, 383–393. https://doi.org/10.1016/j.apenergy.2016.08.128ArticleGoogle Scholar
- Simonson, C. J., & Besant, R. W. (1999a). Energy wheel effectiveness. Part II. correlations. International Journal of Heat and Mass Transfer, 42(12), 2171–2185. ArticleGoogle Scholar
- Simonson, C. J., & Besant, R. W. (1999b). Energy wheel effectiveness: Part I—Development of dimensionless groups. International Journal of Heat and Mass Transfer, 42(12), 2161–2170. https://doi.org/10.1016/S0017-9310(98)00325-1ArticleMATHGoogle Scholar
- Srimuang, W., & Amatachaya, P. (2012). A review of the applications of heat pipe heat exchangers for heat recovery. Renewable and Sustainable Energy Reviews, 16(6), 4303–4315. https://doi.org/10.1016/j.rser.2012.03.030ArticleGoogle Scholar
- Tu, R., Liu, X-H., Jiang, Y. (2013). Performance comparison between enthalpy recovery wheels and dehumidification wheels. International Journal of Refrigeration, 36(8), 2308–2322. Google Scholar
- Vali, A., Simonson, C. J., Besant, R. W., & Mahmood, G. (2009). Numerical model and effectiveness correlations for a run-around heat recovery system with combined counter and cross flow exchangers. International Journal of Heat and Mass Transfer, 52(25), 5827–5840. https://doi.org/10.1016/j.ijheatmasstransfer.2009.07.020ArticleMATHGoogle Scholar
- Wallin, J., Madani, H., & Claesson, J. (2012). Run-around coil ventilation heat recovery system: A comparative study between different system configurations. Applied Energy, 90(1), 258–265. https://doi.org/10.1016/j.apenergy.2011.05.012ArticleGoogle Scholar
- Wang, J. C. Y. (1985). Practical thermal design of run-around air-to-air heat recovery system. Journal of Heat Recovery Systems, 5(6), 493–501. https://doi.org/10.1016/0198-7593(85)90216-4ArticleGoogle Scholar
- Wang, H., Zhou, S., Wei, Z., & Wang, R. (2016). A study of secondary heat recovery efficiency of a heat pipe heat exchanger air conditioning system. Energy and Buildings, 133, 206–216. https://doi.org/10.1016/j.enbuild.2016.09.061ArticleGoogle Scholar
- Xue, L., Ma, G., Zhou, F., & Wang, L. (2019). Operation characteristics of air–air heat pipe inserted plate heat exchanger for heat recovery. Energy and Buildings, 185, 66–75. https://doi.org/10.1016/j.enbuild.2018.12.036ArticleGoogle Scholar
- Yau, Y. H. (2007). Application of a heat pipe heat exchanger to dehumidification enhancement in a HVAC system for tropical climates—A baseline performance characteristics study. International Journal of Thermal Sciences, 46(2), 164–171. https://doi.org/10.1016/j.ijthermalsci.2006.02.006ArticleGoogle Scholar
- Yau, Y. H., & Ahmadzadehtalatapeh, M. (2010). A review on the application of horizontal heat pipe heat exchangers in air conditioning systems in the tropics. Applied Thermal Engineering, 30(2), 77–84. https://doi.org/10.1016/j.applthermaleng.2009.07.011ArticleGoogle Scholar
- Zeng, C., Liu, S., & Shukla, A. (2017). A review on the air-to-air heat and mass exchanger technologies for building applications. Renewable and Sustainable Energy Reviews, 75, 753–774. https://doi.org/10.1016/j.rser.2016.11.052ArticleGoogle Scholar
- Zhang, L. Z., & Jiang, Y. (1999). Heat and mass transfer in a membrane-based energy recovery ventilator. Journal of Membrane Science, 163, 29–38. ArticleGoogle Scholar
- Zhang, L. Z., & Niu, J. L. (2002). Performance comparisons of desiccant wheels for air dehumidification and enthalpy recovery. Applied Thermal Engineering, 22(12), 1347–1367. https://doi.org/10.1016/S1359-4311(02)00050-9ArticleGoogle Scholar
- Zhou, F., Duan, W., & Ma, G. (2018). Thermal performance of a multi-loop pump-driven heat pipe as an energy recovery ventilator for buildings. Applied Thermal Engineering, 138, 648–656. https://doi.org/10.1016/j.applthermaleng.2018.04.104ArticleGoogle Scholar
Acknowledgements
Fundamental Research Grant Scheme, Ministry of Education Malaysia (203/PTEKIND/6711574; 203/PTEKIND/6711274).